Radionuclide monitoring
Radionuclide technology
Radionuclide technology is complementary to the three waveform technologies used in the CTBT verification regime, and the only one that can confirm whether an explosion detected and located by the others is indicative of a nuclear test.
Radionuclide stations measure radioactive particles and noble gases, i.e. radionuclides, in the air. A radionuclide is an isotope with an unstable nucleus that loses its excess energy by emitting radiation in the form of particles or electromagnetic waves in a process called radioactive decay.
Radionuclides – often called radioisotopes – may occur naturally, but they can also be artificially produced. Primordial radionuclides originate mainly from the interiors of stars. Some of them, such as uranium and thorium, decay very slowly and are therefore still present in our universe today. Artificially produced radionuclides can be generated by nuclear reactors, particle accelerators, radionuclide generators or nuclear explosions.
Noble gases are chemical elements that normally occur in their gaseous state. The name 'noble gases' emphasizes the fact that these elements are inert and rarely react with other chemicals. In comparison to radionuclide particles, noble gas atoms are very small.
Like other elements, noble gases also occur in nature in a number of isotopes, some of which are unstable and emit radiation. There are some radioactive noble gas isotopes, i.e. radionuclides, which do not occur naturally but can only be produced by nuclear reactions. Due to their nuclear properties, four isotopes of the noble gas xenon are particularly relevant to the detection of nuclear explosions.
Looking for the 'smoking gun'
Most of the energy of a nuclear explosion is transformed into the immediate blast, shockwaves and heat -- explosive energy that is released within less than a minute. Initial radiation accounts for another small fraction of the energy released during a nuclear explosion. The remaining 10% of the energy is released as residual radiation, which is emitted over time, mainly through radioactive decay of the explosion’s fission products.
Fission products, in solid and gaseous form, are isotopes generated during a nuclear chain reaction. Some of these isotopes are stable. Most are not and undergo radioactive decay, i.e. they are radioactive. Following an atmospheric nuclear explosion, solid fission products attach to dust particles that are propagated by prevailing winds over great distances.
Underwater nuclear explosions also release radioactive particles into the atmosphere. Even shallow underground nuclear explosions can be detected by their release of radioactive debris.
Well-contained underground or deep underwater nuclear explosions, however, do not release any radioactive particles into the air. Another method is needed to detect them.
Radioactive noble gas isotopes – in particular xenon isotopes – are among the fission products generated in a nuclear explosion. Since they are inert, these xenon isotopes will not attach to debris or dust to form larger particles. They remain in their gaseous state and some of them will seep through layers of rock and sediment until they escape into the air. Exposed to prevailing winds, they are dispersed in the atmosphere and may, after a certain period of time, be detected thousands of kilometres away from the explosion site.
The objective of the CTBTO’s radionuclide monitoring network is to detect this residual radiation in the form of radioactive particles or noble gas, even if only in miniscule amounts. By literally collecting and analysing the debris of a nuclear explosion, radionuclide technology is the only one of the four IMS technologies that can provide evidence that an explosion has been nuclear in nature.
Thus, this technology provides the means to identify the 'smoking gun' needed to prove a possible violation of the Treaty. With its 'forensic proof' of nuclear explosions, the radionuclide technology is of crucial importance to the entire verification effort.
However, this does not mean that the CTBTO itself determines whether an explosion has been nuclear in nature or not. It is the prerogative of Member States to make an assessment on the nature of an event, based on monitoring data and analysis provided by the CTBTO.
How the radionuclide monitoring network works
The 80-station radionuclide monitoring network enables a continuous worldwide observation of aerosol samples of radionuclides. The network is supported by 16 radionuclide laboratories with expertise in environmental monitoring, providing independent additional analysis of IMS samples.
Stations must have a denser presence near the equator than in higher latitudes because global wind fields in the equatorial region are virtually vertical, while in the North and South they are more lateral. This means that, in the higher latitudes, radionuclides are transported horizontally most effectively. The more stations there are, the greater the probability of detection and the shorter the probable time period.
In terms of air sampling at the station site, it is advantageous to have a good mixing of surface air with upper layers of air. In principle, the site should be a windy, exposed place where the passing air really hits the sampler used to collect particulates transported by the wind. The larger the air volume, the greater the efficiency for particulate sampling.
One disadvantage with this type of detection is that it is passive, relying on air currents to move the particles or gases to the radionuclide detection site. This is why so many stations are needed for the monitoring of radionuclides.
A radionuclide particulate monitoring station contains an air sampler, detection equipment, computers and a communication setup. Air is forced through a filter, which retains more than 85% of all particles that reach it. Filters are replaced daily. The used filter is first cooled for a period of 24 hours and then measured for another 24 hours in the detection device at the monitoring station. The result is a gamma ray spectrum that is sent to the International Data Centre (IDC) in Vienna for further analysis.
In noble gas monitoring systems, air is pumped into a charcoal-containing purification device where xenon is isolated. Contaminants of different kinds, such as dust, water vapour and other chemical elements are eliminated. The resulting air contains higher concentrations of xenon, both in its stable and unstable (i.e. radioactive) forms. The radioactivity of the isolated and concentrated xenon is measured and the resulting spectrum is sent to the IDC for further analysis.
Data sent by the radionuclide stations to the IDC do not only include gamma radiation spectra, but also meteorological and state-of-health information. State-of-health data provide information on the station’s operational status and the quality of the raw monitoring data it transmits.
Support is provided on a regional basis by 16 radionuclide laboratories, which conduct sample analyses if and when necessary. Their main function is to provide independent analysis of particulate samples suspected of containing radionuclides that may have been produced by a nuclear explosion, and to conduct routine analyses for quality control of a station’s air sample measurements.
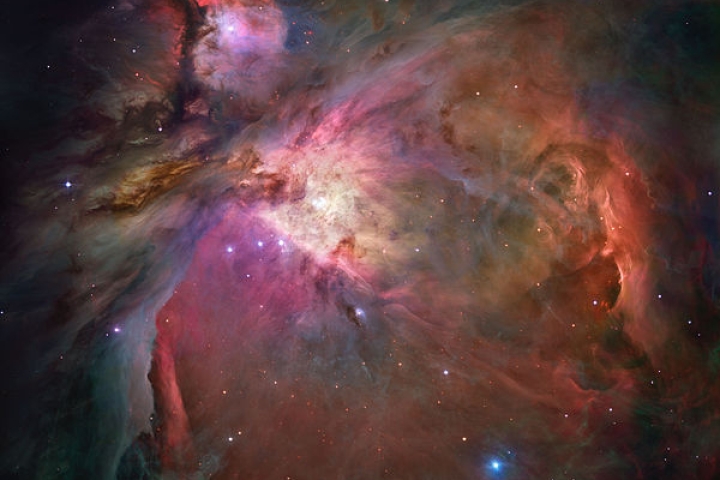
Primordial radionuclides originate mainly from the interior of stars. Here, the Orion Nebula.
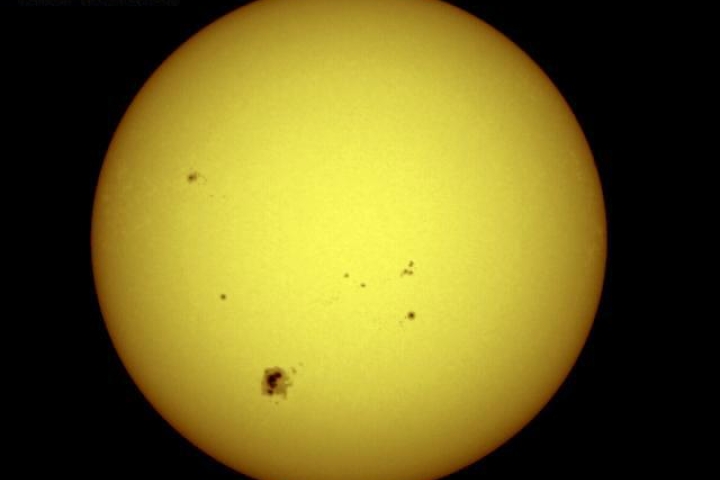
The sun consists in part of the noble gas helium.
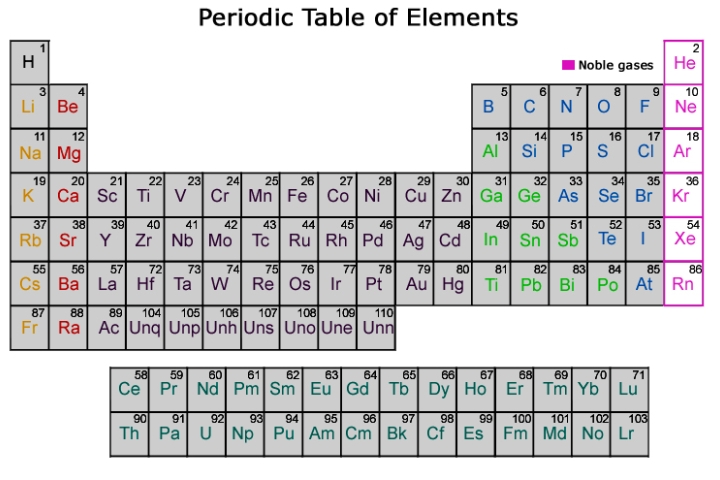
Periodic table of chemical elements.
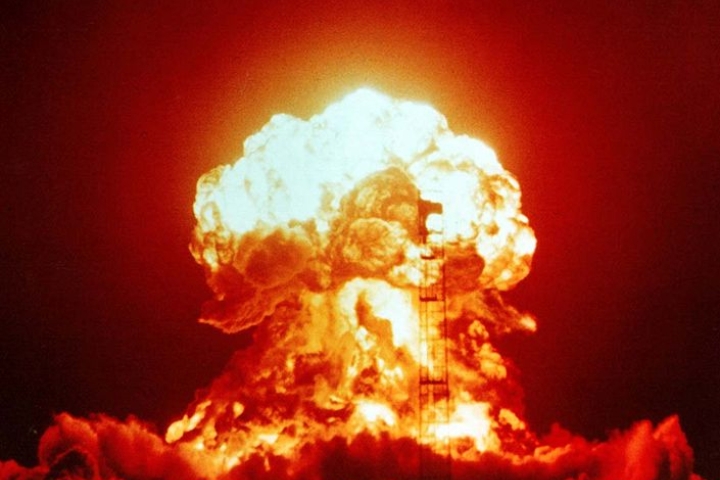
Nuclear test, Operation Upshot-Knothole, Event Badger, 18 April 1953, Nevada Test Site, USA.
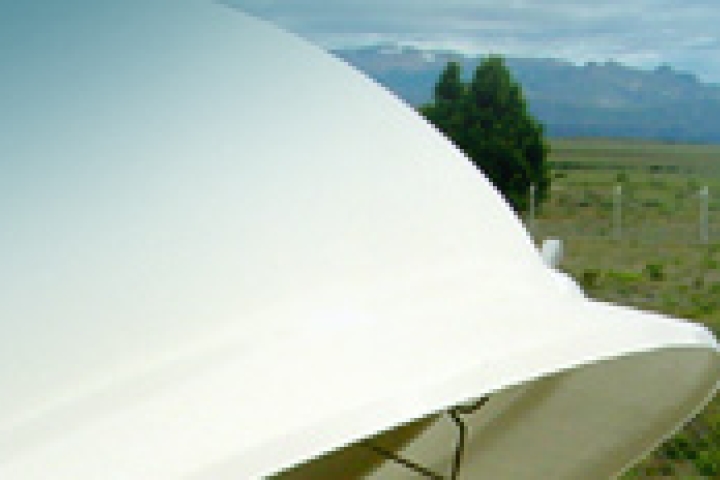
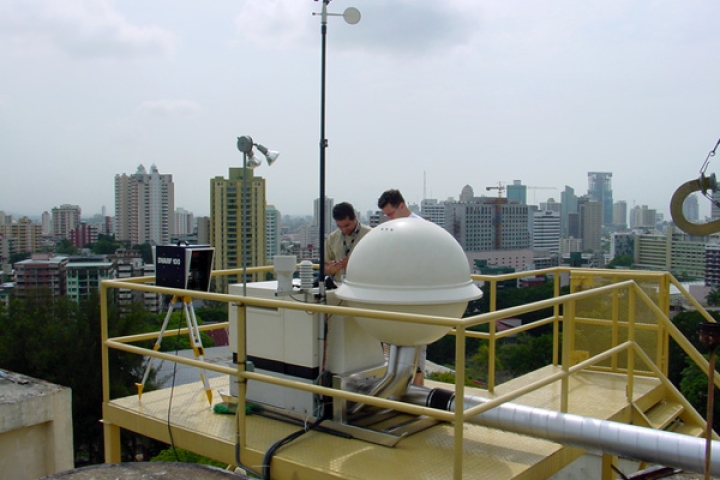
Data from 80 radionuclide station are sent to the IDC for further analysis. Radionuclide station RN50, Panama City, Panama.
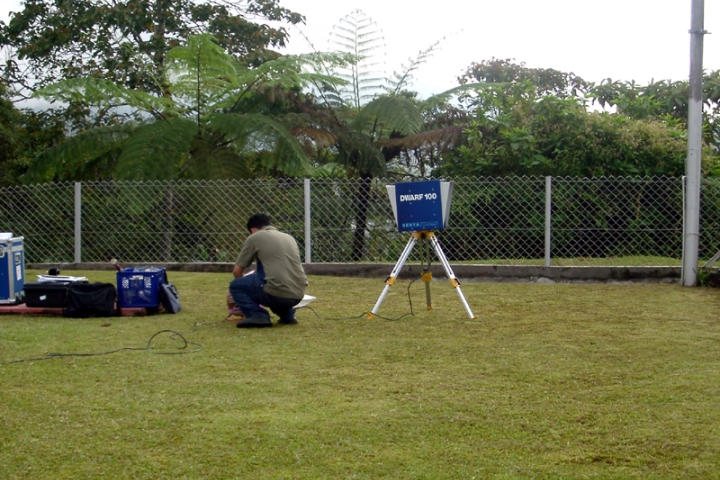
Aerosol sampling during a site survey, radionuclide station RN42, Tanah Rata, Malaysia.
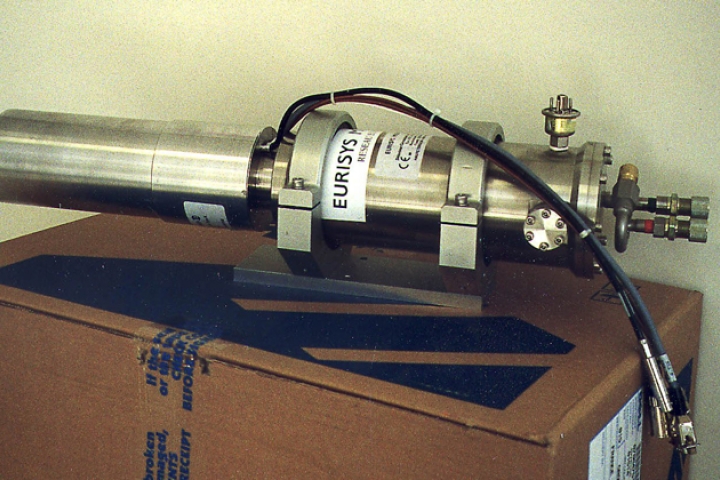
Gamma ray detector.
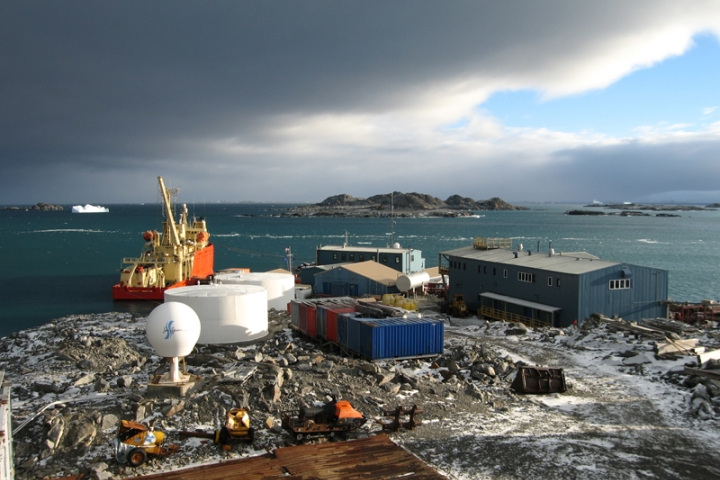
Radionuclide station 73, Palmer station, Antarctica
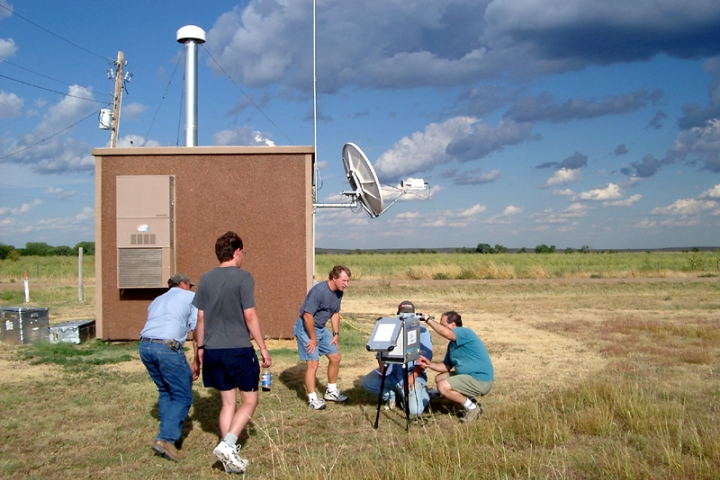
Radionuclide station RN74, Ashland, Kansas, USA.
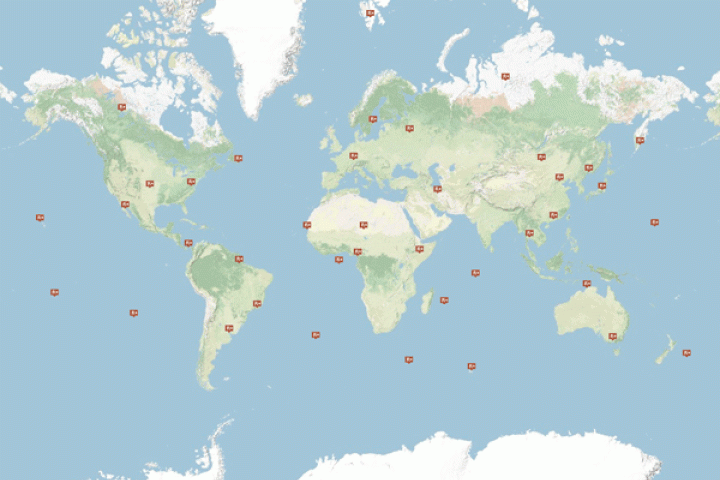
28 of the 40 planned INGE stations were in operation in 17 countries as of March 2011, with three of them being already certified.
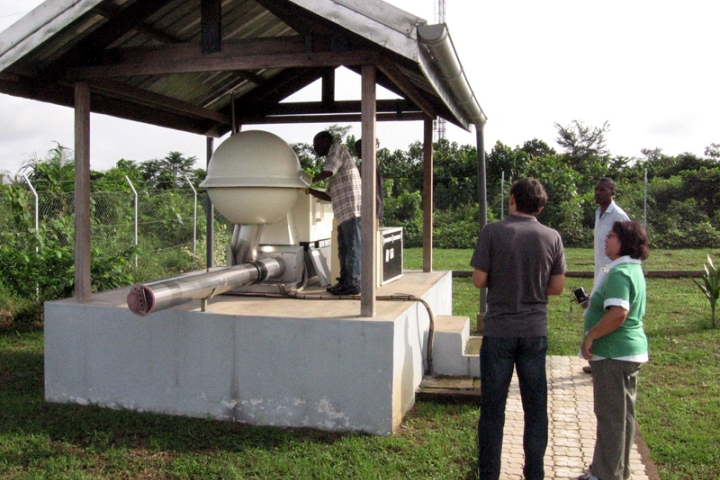
Radionuclide station RN13, Douala, Cameroon.